Gaojian Liu defens his master's thesis
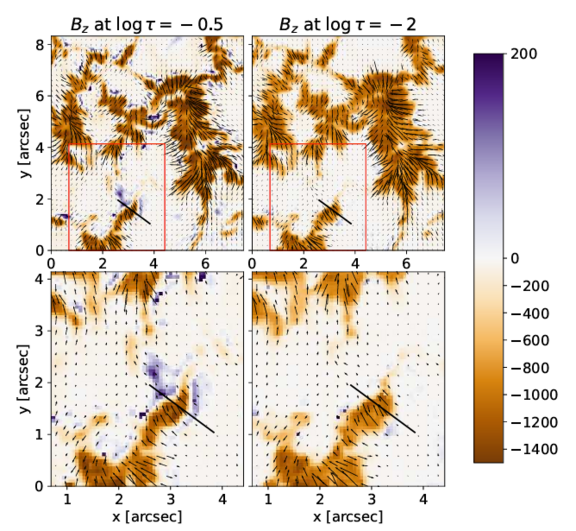
Gaojian Liu, a master's student of physics at the University of Freiburg, succesfully defended his thesis titled: Small-scale Dynamics of a Solar Plage Revealed by Integral Field Spectropolarimetry
Examiner was prof. Marc Schumann, and supervisors of the work were Juan Manuel Borrero and Ivan Milic. Gaojian will continue his work with us in upcoming months and conduct additional analysis of the data. He already published an Astronomy and Astrophysics letter with a part of his findings, specifically the presence of small-scale opposite polarities at plage boundaries (See the figure). Congraulations Gaojian!
Link to the paper:
Abstract of the thesis: The Sun is an astrophysical object of paramount importance both for fundamental science and our civilization. One of the most important aspects of the Sun is its magnetic field, which is structured on a range of spatial and temporal scales. The main source of information about the solar magnetic fields is the observations of the intensity and polarization of specific spectral lines in the solar spectrum. Combining spectropolarimetric observations with high-resolution imaging allows us to probe the structure of the solar atmosphere in all three dimensions, and if the observations are taken at multiple instances in time. In this thesis, we analyze a unique dataset of this kind: high spectral, spatial, and temporal resolution observations of a small segment of a solar plage, obtained with a prototype integral field unit called Microlensed Hyperpectral Imager (MiHI).
The thesis describes the theoretical framework needed to interpret this data, the observational and data reduction techniques, and presents some findings related to the plage structure and dynamics. The inferred plage structure and its statistical properties agree very well with previous studies. Moreover, the plage contains very small-scale opposite polarities which have not been fully resolved before. The fast cadence of MiHI allowed us to monitor these opposite polarities in time. We conclude that these opposite polarities change slightly with time but do not exhibit fundamental changes like emergence, submergence, or merging.
Jan Buttler defens his bachelor thesis
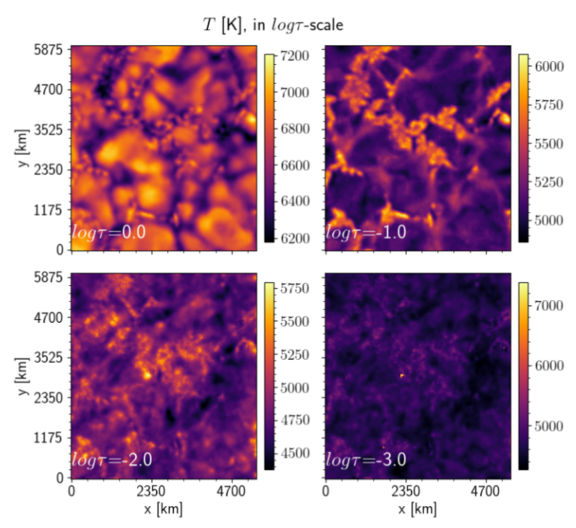
Jan Buttler, a bachelor student of physics at the University of Freiburg, succesfully defended his bachelor thesis titled: Inference of the 3-D Structure of a Solar Plage in the Sodium I D1 line
Examiner was prof. Marc Schumann, and supervisors of the work were Juan Manuel Borrero and Ivan Milic. Jan will continue his work with us in upcoming months and work toward publishing his work in a journal. Congratulations Jan!
Abstract of the thesis: The Sun is a natural plasma laboratory - due to its vicinity, it can be observed with very high spatial, spectral, and temporal resolution, using spectropolarimetry. These observations can be used to better understand the interaction between plasma, radiation, and magnetic field. However, to interpret the spectropolarimetric observations of the Sun, we must first understand the microscopic interaction between the light and the atoms that absorb, emit, and scatter the light, as well as the effects that cause polarization in the observed spectral lines. This thesis describes such spectropolarimetric observations and outlines the theoretical and numerical tools we use to interpret them. Furthermore, it showcases an interpretation of a unique dataset: integral field unit observations, obtained using the recently developed MiHI instrument mounted at the Swedish Solar Telescope. The data contains spectropolarimetric observations of the Fraunhofer D1 line of neutral sodium. To interpret these observations we use a numerical code called FIRTEZ, which is a so-called inversion-code, that can retrieve physical parameters from spectropolarimetric observations. Our results show that this spectral line carries information about the vertical magnetic field throughout the photosphere and that, thanks to the unique capabilities of FIRTEZ, we can reconstruct the structure of this vertical field with height in geometrical $z$-scale. However, information on the horizontal component of the field is unavailable, due to the limited signal-to-noise ratio of the data, even after temporal binning. Finally, the inferred velocities in the upper photosphere show an interesting fibril-like structure.
KIS in ARD news in prime time
On the Christmas eve, 2024-Dec-24, Parker Solar Probe had a closest Sun encounter; KIS scientist, Gregory Fleishman, commented on that in the main national News on ARD.
Watch the episode dedicated to this event here:
Comparing acoustic flux with the chromospheric radiative losses using NLTE inversions
An open question in the physics of the lower solar atmosphere is how the chromosphere gets the energy it needs to sustain the radiative losses. One often suggested option is that the acoustic waves supply the energy through wave dissipation. This study, led by João da Silva Santos (NSO) and Momchil Molnar (HAO, now SWRI), which also involved Ivan Milic from KIS, investigated this using a detailed NLTE inversion of multiple photospheric and chromospheric spectral lines observed using IBIS and IRIS instruments. The study utilized state-of-the-art inversion code StIC, combined with machine learning techniques to make interpretation of these large datasets feasible. The techniques to characterize acoustic flux and radiative losses were verified and calibrated on chromospheric MHD simulations, before being applied to the observation. The general conclusion of the paper is that, while the inversions do overestimate radiative losses and underestimate acoustic fluxes - even after the correction, the acoustic flux does not seem to provide enough energy to heat the quiet Sun chromosphere.
The study is published in the Astrophysical Journal:
iopscience.iop.org/article/10.3847/1538-4357/ad81d4/pdf
New DFG proposal funded at the Solar Magnetism group
The German Science Foundation (DFG) has recently funded a proposal by Dr. Gregory D Fleishman, the Leader of the Solar Magnetism group at KIS. The proposal, MIRACLE: Magnetic Interactions Releasing Acceleration in Coronal-flaring Loop Environment, is funded for a period of three years to support one postdoc FTE and aims at improving our ability to measure and model coronal magnetic field and study associated phenomena. The project will obtain new fundamental knowledge on magnetic reconnection and particle acceleration in solar flares via (i) measuring the coronal magnetic field and its variations; (ii) measuring the nonthermal electron distributions and evaluating a typical efficiency of acceleration; and (iii) understanding these dynamics in the 3D domain. The project will combine the remote sensing of the evolving coronal magnetic field and other parameters in solar flares with data-constrained 3D modeling. The project will yield evolving microwave images of solar flares and active regions, evolving maps of coronal parameters such as magnetic field (see Figure) and plasma densities, new physical phenomena derived from these data and data products, and 3D models of these phenomena. Reviewers were extremely enthusiastic about the project: “This is a compelling, well-written, and impactful project by a world-renowned expert in the field. It has a clear potential for breakthroughs in our understanding of the physics of solar flares, perhaps the most important and influential manifestations of solar activity.”
Comparison betwen MHD simulations and observations of the magnetic field in the solar internetwork.
The solar internetwork occupies most of the solar surface. In these regions the magnetic field is very weak and consequently the observed polarization signals are very low and barely above the noise level. Because of this, inferences of the magnetic field via Stokes inversion techniques are prone to error and result in magnetic fields that are too horizontal. In order to avoid these shortcomings we have performed a statistical study of observed polarization signals in the internetwork observed with the Hinode/SP instrument at many different heliocentric positions on the solar disk. These observed signals are compared with the predictions by MHD simulations carried out with different boundary/initial conditions: a) small-scale dynamo simulation; b) simulation with initial vertical magnetic fields; c) emergence of horizontal field from the bottom boundary. Out of these three simulations, the small-scale dynamo simulation fits better the histograms of the observed signals at different heliocentric angles (see orange lines in the figure above). However, even for this simulation the agreement is not perfect. We suggest that it could be improved if the magnetic field in the MHD simulation would became less horizontal in the higher photospheric layers.
This work has been accepted for publication in Astronomy & Astrophysics: Ebert, Milic & Borrero, A&A, in press.
Mike Moser sucessfully defends his MSc on "Investigation of super-strong magnetic Fields in Sunspots using GREGOR Infrared Data"
Mike Moser has been a MSc student at our institute under the supervision of Dr. Borrero, Dr. Milic and Prof. von der Lühe since October 2023. On October 17th, 2024 Mr. Moser successfully defended his MSc thesis on the topic of “Investigation of super-strong magnetic Fields in Sunspots using GREGOR Infrared Data". His MSc thesis can be found here.
In this thesis Mike has studied the magnetic field in several counter Evershed flow regions in three different sunspots observed with the GRIS instrument attached to 1.5-meter GREGOR Telescope. The spectropolarimetric data contains several Fe I spectral lines in the wavelength region around 1565 nm. These spectral lines are extremely sensitive to the magnetic field in the deep photosphere. The data have been analyze employing the FIRTEZ inversion code while self-consistently considering the effect of the telescope's point spread function. This technique allows to successfully reproduce multi lobes Stokes profiles. Unlike previous studies using less sensitive spectral lines, these new results do not show the presence of magnetic fields above 3000 Gauss in the deep Photosphere in counter Everflow regions (see rightmost panel in figure above).
Giant Post-Flare Loops in Active Regions with Extremely Strong Coronal Magnetic Field
An international team of researchers (KIS: Gregory Fleishman) reports for the first time the detection of thermal free-free emission from large post-flare loops at 34 GHz in images from the Nobeyama Radioheliograph (NoRH). 8 loops were studied, 7 of which were from regions with extremely strong coronal magnetic field reported in https://ui.adsabs.harvard.edu/abs/2023ApJ...943..160F/abstract. Loop emission was observed in a wide range of wavelength bands, up to soft X-rays, confirming their multi-temperature structure and was associated with noise storm emission in metric waves. The comparison of the 17 GHz emission with that at 34 GHz showed that the emission was optically thin at both frequencies. The computed density at the top of the loops is between 1 and 6×1010 cm-3, noticeably varying from one loop to another and in the course of the evolution of the same loop system These density values are above those reported from EUV observations, which go up to about 1010 cm-3. This difference could be due to the fact that different emitting regions are sampled in the two domains and/or due to the more accurate diagnostics in the radio range, which do not suffer from inherent uncertainties arising from abundances and non-LTE excitation/ionization equilibria. We also estimated the magnetic field in the loop tops (roughly, 100 Mm above the solar surface) to be in the range of 10-30 G, which adds new data points to a reference plot of the coronal magnetic field vs height (see the figure).
ApJ; published: https://ui.adsabs.harvard.edu/abs/2024arXiv240614638A/abstract
See the interactive plot at: www.leibniz-kis.de/de/forschung/sonnenphysik/koronales-magnetfeld/
INTEGRAL Picture-of-the-Month in August 2024 is Selected from a KIS Publication
A recent KIS paper https://ui.adsabs.harvard.edu/abs/2024A%26A...686A.195K/abstract (see below in this page) employed high-energy INTEGRAL data to reveal “coupling between magnetic reconnection, energy release, and particle acceleration in solar flares.” Figure 2 from this paper was called INTEGRAL Picture-of-the-Month (POM). Solar flares are energetic transient phenomena that involve several phases of energy transformation. After a complex preflare buildup of the free magnetic energy in the solar corona, an instability leads to a sudden release of the free energy during a flare by magnetic reconnection driven by strong, likely turbulent, plasma flows and accompanied by a strong dynamic electric field. The tracers of this released energy are the emission observed from radio to gamma-rays, solar energetic particles, and coronal mass ejections. A significant portion of the energy is spent on the acceleration of charged particles and plasma heating, which includes heating due to the collisional energy loss of the flare-accelerated particles.
In this collaborative study (KIS: Gregory Fleishman), the international team of researchers addressed a question of relationships and coupling between the magnetic reconnection, energy release and acceleration of charged particles up to highest energies in one of the strongest ever recorded X17.2 2003 October 28 solar flare. The spectral analysis of the high-energy γ-ray emission revealed a close association between the acceleration process efficiency and the reconnection rates. High-energy bremsstrahlung continuum and narrow γ-ray lines were observed in the main rise phase when the reconnection electric field reached values of ∼120 V cm−1. In the main energy release phase, the upper energy of the bremsstrahlung spectrum was significantly reduced and the pion-decay γ-ray emission appeared abruptly. We argue that the main energy release and proton acceleration up to subrelativistic energies began just when the reconnection rate was going through the maximum i.e., after a major change of the flare topology.
AA (accepted): https://arxiv.org/abs/2403.08135
The full POM archive can be found here:
https://www.cosmos.esa.int/web/integral/pom-archive
Electron Cyclotron Maser Emission and the Brightest Solar Radio Bursts
An international team of collaborators (KIS: Gregory Fleishman) investigates the incidence of coherent emission in solar radio bursts, using a revised catalog of 3800 solar radio bursts observed by the Nobeyama Radio Polarimeters from 1988 to 2023. We focus on the 1.0 and 2.0 GHz data, where radio fluxes of order 1010 Jansky have been observed. Previous work has suggested that these bursts are due to electron cyclotron maser (ECM) emission. In at least one well-studied case, the bright emission at 1 GHz consists of narrowband spikes of millisecond duration. Coherent emission at 1 GHz can be distinguished from traditional incoherent gyrosynchrotron flare emission based on the radio spectrum: gyrosynchrotron emission at 1 GHz usually has a spectrum rising with frequency, so bursts in which 1 GHz is stronger than higher frequency measurements are unlikely to be incoherent gyrosynchrotron. Based on this criterion it is found that, for bursts exceeding 100 sfu, three-quarters of all bursts at 1 GHz and half of all 2 GHz bursts have a dominant coherent emission component, assumed to be ECM. The majority of the very bright bursts at 1 GHz are highly circularly polarized, consistent with a coherent emission mechanism, but not always 100% polarized. The frequency range from 1 to 2 GHz is heavily utilized for terrestrial applications, and these results are relevant for understanding the extreme flux levels that may impact such applications. Further, they provide a reference for comparison with the study of ECM emission from other stars and potentially exoplanets.
ApJ: https://ui.adsabs.harvard.edu/abs/2024ApJ...969....3W/abstract
New DFG proposal funded at the Solar Magnetism group
The German Science Foundation (DFG) has recently funded a proposal by Dr. Juan Manuel Borrero (P-I) and Dr. Ivan Milic (Co-I) in the Solar Magnetism group. The proposal, Advacing Currents Determination in the Chromosphere or ACDC, will be funded for a period of three years and aims at improving our ability to forecast explosive and energetic events in the solar atmosphere by measuring the electric current vector in the solar Photosphere and Chromosphere. Electric currents are produced by non-potential magnetic fields that are known to be highly unstable configurations. As such, they are prone to sudden changes in the connectivity of the field lines towards a lower energy state (potential configuration). The energy difference between the initial non-potential (high energy state) and final potential (low energy state) is released into plasma via a process called magnetic reconnection. Magnetic reconnection is responsible for phenomena such as flares and coronal mass ejections (see figure on the right) that can affect the upper layers of Earth's atmosphere and therefore forecasting such phenomena is a high priority for solar physicists world-wide and in particular for our group at the Institute for Solar Physics.
The inference of electric currents will be done via the analysis of spectropolarimetric data with modern observing facilities: our 1.5-meter solar telescope GREGOR, the VTF instrument attached to the 4-meters DKIST solar telescope, and the Sunrise stratospheric balloon. The analysis will be carried out with an improved version of the FIRTEZ inversion code that will account for Non-Local Thermodynamic Equilibrium effects (NLTE) as well as for the induction equation that links the velocitiy of the solar plasma with the time variation of the magnetic field on its surface.
Solar Jet Hunter: a citizen science initiative to identify coronal jets in EUV data sets
Solar coronal jets seen in EUV are ubiquitous on the Sun, have been found in and at the edges of active regions, at the boundaries of coronal holes, and in the quiet Sun. Jets have various shapes, sizes, brightness, velocities and duration in time, which complicates their detection by automated algorithms. So far, solar jets reported in the Heliophysics Event Knowledgebase (HEK) have been mostly reported by humans looking for them in the data, with different levels of precision regarding their timing and positions. To radically improve the jet detection, an international team of collaborators (KIS: Gregory Fleishman) designed a citizen science project, "Solar Jet Hunter", on the Zooniverse platform, to analyze EUV observations at 304 Å from the Solar Dynamic Observatory/Atmospheric Imaging Assembly (SDO/AIA). This project created a catalogue of solar jets observed in EUV at 304 Å that contains precise and consistent information on the jet timing, position and extent. To do so, hundreds of citizen scientists (volunteers) analyzed more than 120,000 images distributed into 9,689 "movie strips" from 2011 to 2016 and reported the timing, position and extent of the 883 identified individual jet. We demonstrate how citizen science can enhance the analysis of solar data with the example of Solar Jet Hunter. The catalogue of jets thus created is publicly available at https://conservancy.umn.edu/handle/11299/257209 and will enable statistical studies of jets and related phenomena. This catalogue will also be used as a training set for machines to learn to recognize jets in further data sets.
AA (accepted): https://arxiv.org/pdf/2309.14871
First Stokes inversions in full agreement with Gauß law
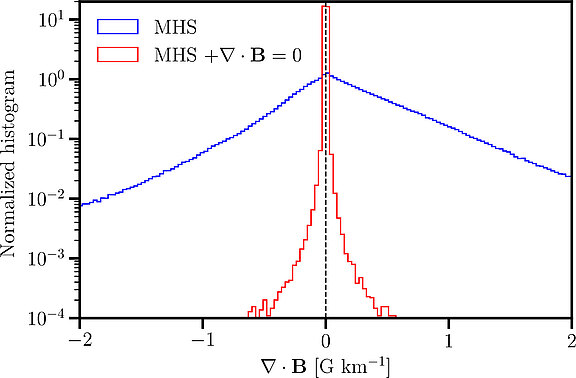
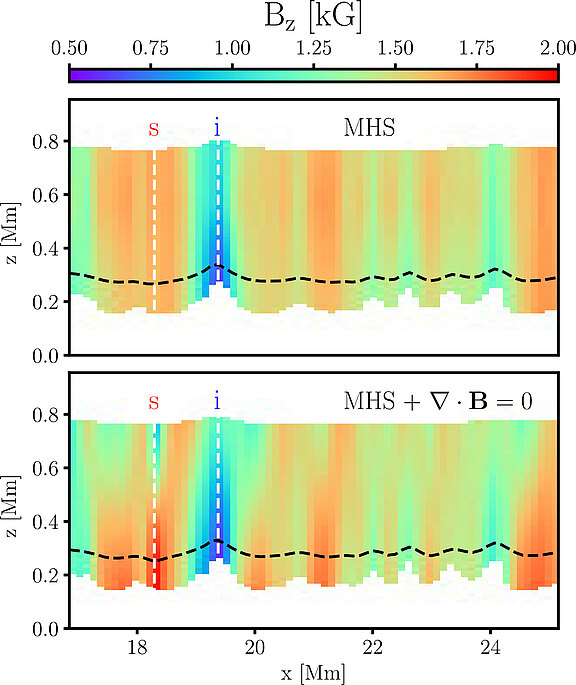
Gauß law for magnetism implies that the magnetic field must be solenoidal: ∇ B = 0. This is also known as Maxwell's second equation. Unfortunately, traditional Stokes inversion codes for solar spectropolarimetric data retrieve a magnetic field B that is non-solenoidal and thus in direct disagreement with one of the most important equations of classical electrodynamics. Researchers at KIS (J.M. Borrero) have led an international team, including Adur Pastor Yabar (Stockholm University, Sweden) and Basilio Ruiz Cobo (Instituto de Astrofisica de Canarias, Spain) in an effort to determine, for the very first time, a magnetic field on the solar atmosphere that is fully compatible with the solenoidal condition. This opens the possibility to dramatically increase the accuracy in the determination of the solar magnetic field. The new method has been implemented into the FIRTEZ inversion code and it is publicly available for the entire community to use through our gitlab page: gitlab.leibniz-kis.de/borrero/firtez-dz-mhs
The work has been accepted for publication in Astronomy and Astrophysics and is available in ArXiv: arxiv.org/abs/2404.02045
Coupling between magnetic reconnection, energy release, and particle acceleration in solar flares
Solar flares are energetic transient phenomena that involve several phases of energy transformation. After a complex preflare buildup of the free magnetic energy in the solar corona, an instability leads to a sudden release of the free energy during a flare by magnetic reconnection driven by strong, likely turbulent, plasma flows and accompanied by a strong dynamic electric field. The tracers of this released energy are the emission observed from radio to gamma-rays, solar energetic particles, and coronal mass ejections. A significant portion of the energy is spent on the acceleration of charged particles and plasma heating, which includes heating due to the collisional energy loss of the flare-accelerated particles.
In this collaborative study (KIS: Gregory Fleishman), the international team of researchers addressed a question of relationships and coupling between the magnetic reconnection, energy release and acceleration of charged particles up to highest energies in one of the strongest ever recorded X17.2 2003 October 28 solar flare. The spectral analysis of the high-energy γ-ray emission revealed a close association between the acceleration process efficiency and the reconnection rates. High-energy bremsstrahlung continuum and narrow γ-ray lines were observed in the main rise phase when the reconnection electric field reached values of ∼120 V cm−1. In the main energy release phase, the upper energy of the bremsstrahlung spectrum was significantly reduced and the pion-decay γ-ray emission appeared abruptly. We argue that the main energy release and proton acceleration up to subrelativistic energies began just when the reconnection rate was going through the maximum i.e., after a major change of the flare topology.
References:
AA (accepted): arxiv.org/abs/2403.08135
Effects of telescope PSF on the magnetic field measurements
Magnetic field distribution in space and time in the solar atmosphere cannot be measured directly. Rather, we infer it from the spatially and spectrally resolved measurements of the intensity and polarization of the light passing through the solar atmosphere. This inference process, called spectropolarimetric inversion, is influenced by the limitations of our instruments.
In this study, led by Ivan Milic (KIS) and Rebecca Centeno (HAO), it has been shown that the spatial resolution of the telescope severely influences our knowledge of the magnetic field. Namely, retrieving intrinsic properties of the field (magnitude, inclination, azimuth) is nigh impossible, even with using ad-hoc parameters (filling factor), and further exacerbated for the observations close to the solar limb (i.e. polar observations). Furthermore, the spatial resolution of the telescopes can significantly influence even spatially averaged parameters (e.g. mean magnetic flux density), which can have potential deep implications for the so-called solar open flux problem.
References:
Milic, Centeno, et al. (A&A in press): https://arxiv.org/abs/2402.02486
Centeno, Milic, et al. (published in ApJ): https://iopscience.iop.org/article/10.3847/1538-4357/acd178/meta
NLTE inversion and modeling workshop in Porto, September, 2023
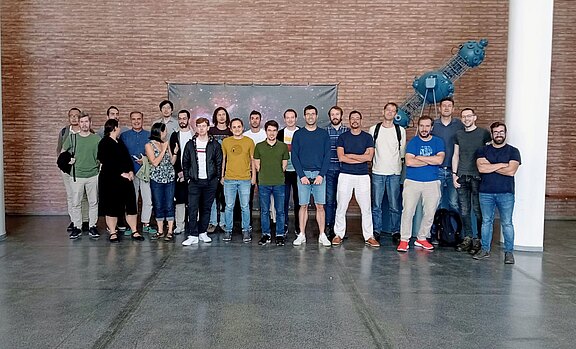
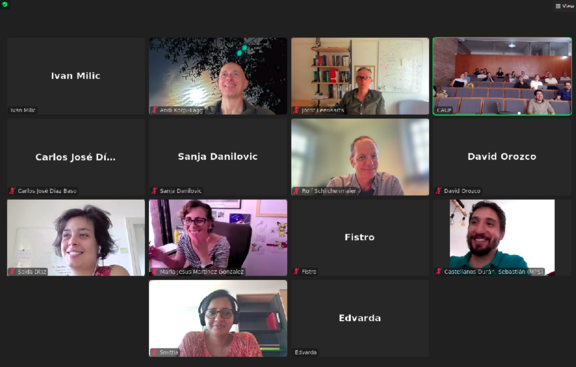
Workshop about NLTE modeling and inverse problems, organized by Ivan Milic (KIS) and Ricardo Gafeira (IA), took place in Porto from 4th to 6th September, 2023. NLTE (non-local thermodynamic equilibrium) is a state of solar atmosphere in which excitation and ionization state of the gas decouple from the local physical conditions (e.g. temperature). This influences the spectral line formation, and in turn the interpretation of the observed spectral lines and their polarization. It is especially relevant for understanding the solar chromosphere, filaments and prominences.
The aim of the workshop was a comprehensive presentation and comparison of the codes for the interpretation of the spectral lines formed in NLTE. It gathered around 40 researchers from Europe and USA, who presented their work and discussed the state of the art. The workshop will be followed up with a research paper on the code comparison and a short white paper on importance of these methods and their development.
Two KIS scientists (Juan Manuel Borrero and Ivan Milic) presented their work, while four more attended the talks and discussions.
Sunspot observation at a spatial resolution of 0.08 acrsec with GREGOR
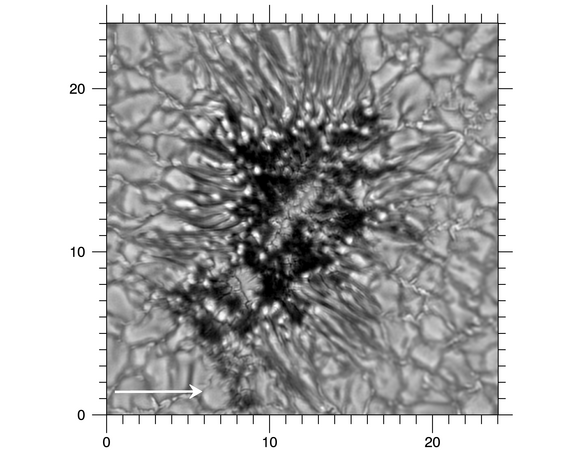
The various mechanisms of magneto-convective energy transport determine the structure of sunspots. Using speckle-reconstructed images in the 486 nm band, we characterise the appearance of light bridges.
We find branched dark lanes extending along thin (≈1′′) light bridges in sunspots at various heliocentric angles. In thick (>2′′) light bridges the branches are disconnected from the central lane and have a Y shape with a bright grain toward the umbra. The images reveal that light bridges exist on varying intensity levels and that their small-scale features evolve on timescales of minutes. Faint-light bridges show dark lanes outlined by the surrounding bright features. Dark lanes are very common and are also found in the boundary of pores. They have a characteristic width of 0.1′′ or smaller. Intergranular dark lanes of that width are seen in active region granulation. We interpret our images in the context of magneto-convective simulations and findings: While central dark lanes in thin light bridges are elevated and associated with a density increase above upflows, the dark lane branches correspond to locations of downflows and are depressed relative to the adjacent bright plasma. Thick light bridges with central dark lanes show no projection effect. They have a flat elevated plateau that falls off steeply at the umbral boundary. There, Y-shaped filaments form as they do in the inner penumbra. This indicates the presence of inclined magnetic fields, meaning that the umbral magnetic field is wrapped around the convective light bridge.
KIS operates and maintains along with its German partners (MPS and AIP) the GREGOR Telescope.
Data-constrained 3D Solar Modeling
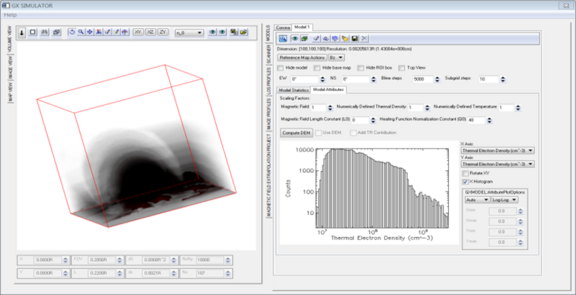
GX Simulatoris an IDL package that combines 3D magnetic and plasma structures with thermal and nonthermal models of the chromosphere, transition region, and corona. Its object-based modular architecture, which runs on Windows, Mac, and Unix/Linux platforms, offers the ability to either import 3D density and temperature distribution models, or to assign numerically defined coronal or chromospheric temperatures and densities, or their distributions, to each individual voxel. GX Simulator can apply parametric heating models involving average properties of the magnetic field lines crossing a given voxel, as well as compute and investigate the spatial and spectral properties of radio–both thermal and nonthermal, (sub)millimeter, EUV, and X-ray emissions calculated from the model, and quantitatively compare them with observations. The package includes a fully automatic model production pipeline that, based on minimal users input, downloads the required SDO/HMI vector magnetic field data, performs potential or nonlinear force-free field extrapolations, populates the magnetic field skeleton with parameterized heated plasma coronal models that assume either steady-state or impulsive plasma heating, and generates non-LTE density and temperature distribution models of the chromosphere that are constrained by photospheric measurements. The standardized models produced by this pipeline may be further customized through specialized IDL scripts, or a set of interactive tools provided by the graphical user interface.
References:
Studying the Poynting flux in the quiet Sun
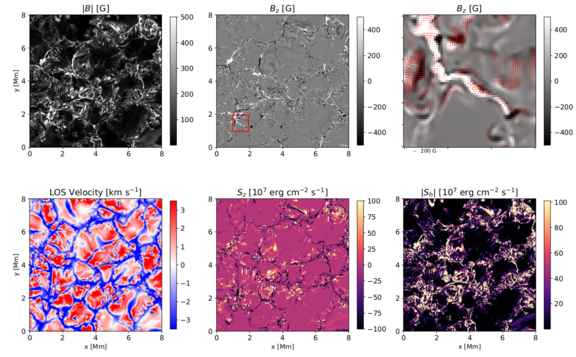
One of the conduits for the energy transfer from the lower to the upper solar atmosphere is the Poynting flux, the vector product of the magnetic and electric field. Estimating the Poynting flux in the quiet Sun is a challenging open problem, because of the low polarization signals that make estimations of these fields extremely uncertain. In this work, the authors analyze SUNRISE/IMAX observations and state-of-the-art numerical simulations to try to estimate the amount of this flux in the solar photosphere, but also to characterize the biases our methods are suffering from due to the limitations of our methods. They combine spectropolarimetric inversions, machine learning methods, and state-of-the-art electric field inference, to try and estimate energy transfer due to the Poynting flux. This is one of the first attempts of this kind and the results are encouraging, but also bring a lot of unanswered questions.
KIS contributes to the SUNRISE balloon-borne observatory with the Correlation and Wavefront Sensor (CWS):
Inferring of the electric current vector in the solar photosphere
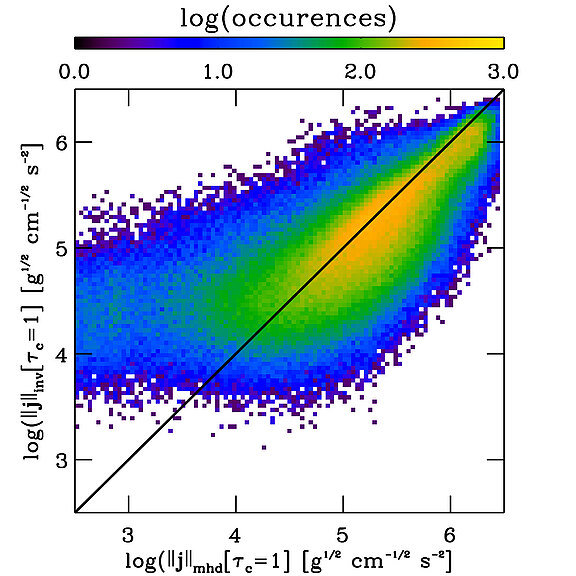
Electric currents play a very important role in the energy balance and transport within the plasma in the solar atmosphere and in particular in the solar corona. They are also proxies for magnetic reconnection which is considered to be the main driver of explosive and transient evens in the chromoshere and corona. Consequently, one of the main goals for the solar physics community has been to measure these electric currents. Despite their importance, routine and direct measurements of electric currents j in the solar atmosphere have not been generally possible. With our new inversion code FIRTEZ-DZ, developed at our institute, we are capable of accurate inferences of the full electric current vector j. This is possible because FIRTEZ considers magneto-hydrostatic equilibrium (MHS) instead of the widely used hydrostatic equilibrium (HE) employed by other inversion codes.
Reference: Pastor Yabar, A.; Borrero, J.M.; Quintero-Noda, C.; Ruiz Cobo, B. (2021), A&ALetters, 656, L20
Sunspot decay
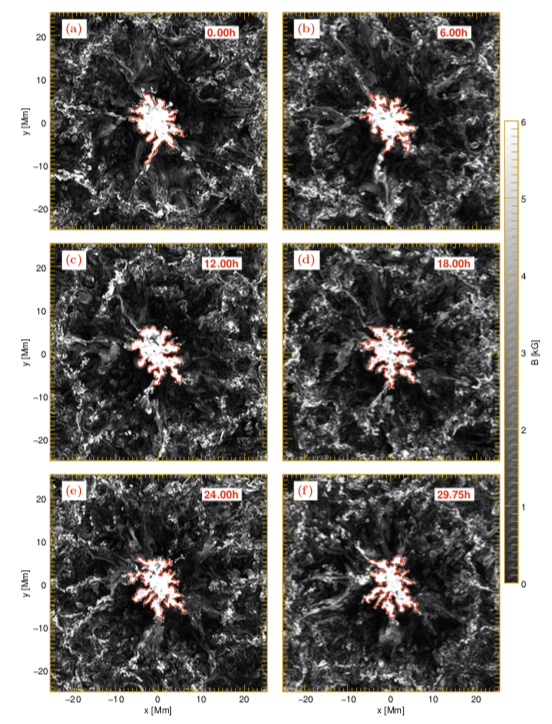
The stability of sunspots is one of the long-standing unsolved puzzles in the field of solar magnetism and the solar cycle. The thermal and magnetic structure of the sunspot beneath the solar surface is not accessible through observations, thus processes in these regions that contribute to the decay of sunspots can only be studied through theoretical and numerical studies.
New insights were gained taking advantage of a numerical simulation of a sunspot with a lateral extension of more than 98 000 km times 98 000 km extending almost 18 000 km beneath the solar surface. These simulations had been performed by Matthias Rempel at the High Altitude Observatory (HAO). A data set of 30 hours, showing a stable sunspot at the solar surface, was analysed with respect to sunspot decay as a PhD project at KIS, in collaboration with the HAO.
This simulation allows to study the non-local dynamics of fluting instability interacting with stabilising buoyance forces within in the magnetic flux tube that constitutes the spot. The simulation shows that the fluting instability causes a corrugation along the circumference of the flux tube (sunspot) which proceeds fastest at a depth around 7500 km beneath the solar surface. For a long time, the decay of the spot is suppressed by the stabilising effect close to the surface which holds the bundle of magnetic field lines together. Yet, ultimately, large intrusions of field-free plasma below the surface form convective bubbles which might form light-bridges in the spot at the solar surface. This can finally disrupt the spot.
The analysis of the simulation also allow to assess the results of a linear stability analysis developed in the 1970s (Meyer, Schmidt, & Weiss 1977). As predicted back then, the magnetic field configuration of a sunspot in the uppermost few 1000 km is stable, mostly due to buoyancy forces. Deeper layers in the spot are unstable to fluting (interchange) instability as it was predicted.
References:
Microwave imaging spectroscopy
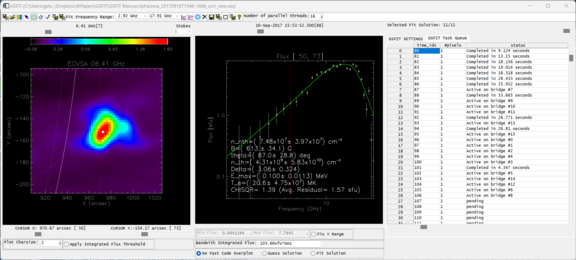
Broadband microwave imaging spectroscopyoffers an entirely new perspective for inferring the evolving coronal parameters of solar flares from microwave observations. These data are becoming increasingly accessible as a routine diagnostics method due to recent technological advances that facilitated the design and development of a new generation of radio instruments, out of which Expanded Owens Valley Solar Array (EOVSA) currently stands out as a world-leading solar dedicated broadband microwave interferometry array. EOVSA features simultaneous imaging at 451 science channels from 1–18GHz, with 1 s cadence. This technique provides a detailed microwave spectrum to be derived at any location of a snapshot image (see Figure on the right). The shape and brightness values of such spectra contain unique fingerprints of the underlying source parameters, such as the magnetic field strength B and the power-law slope $\delta$ of the energetic electron distribution displayed in the spectral plot insets. To facilitate the analysis of the broadband microwave imaging spectroscopy data that led to these breakthrough scientific results, the Dr. Fleishman’s team developed the core methodology, as well as user-friendly analysis (GSFIT) and visualization (GSFITVIEW) tools, which were employed to derive evolving physical parameters in the flare volume.
Polar Magnetic Fields
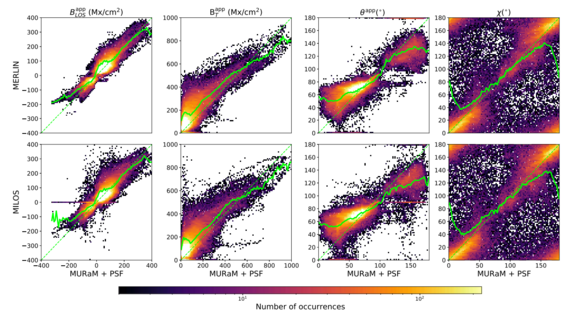
Polar magnetic fields play a key role in the solar cycle and are a main driver of the solar wind. However, characterizing these fields is extremely difficult due to an array of reasons: projection effects, Zeeman effect biases, noise, and magnetic field disambiguation are just some of the problems. To address these biases, a team consisting of Xudong Sun (University of Hawai’i), Rebecca Centeno (HAO), and Ivan Milic (KIS), is conducting a series of end-to-end studies to identify and characterize the biases encountered in the magnetic field estimation process (i.e. the inversion). In the first paper in the series, the authors explore the limits of standard Milne-Eddington inversion and particularly the usage of the filling factor as a free parameter. The results are illuminating, but also bleak: even inference of the “apparent” magnetic field quantities at the poles suffers from large systematic uncertainties. In the upcoming publications, authors are studying individual biases and ways to mitigate them.
Signatures of Retraction and Reconnection in realistic MHD simulations
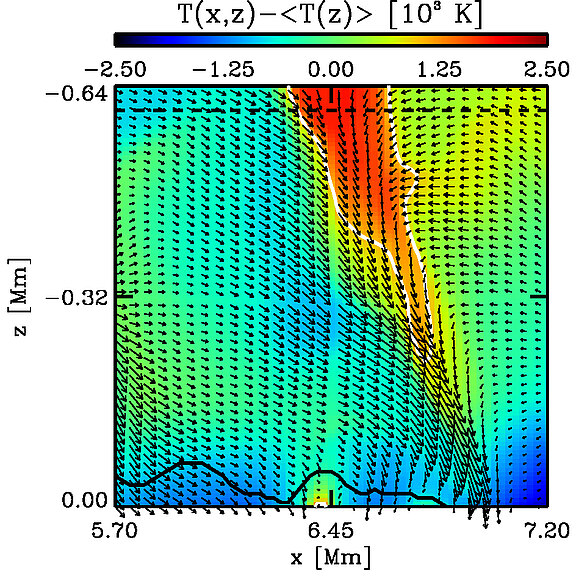
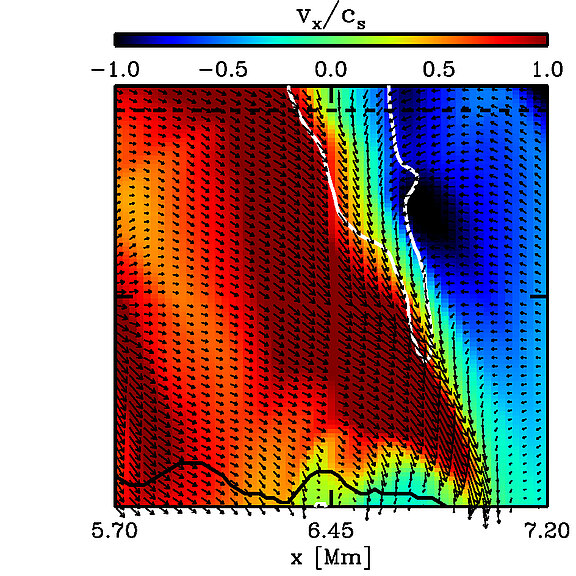
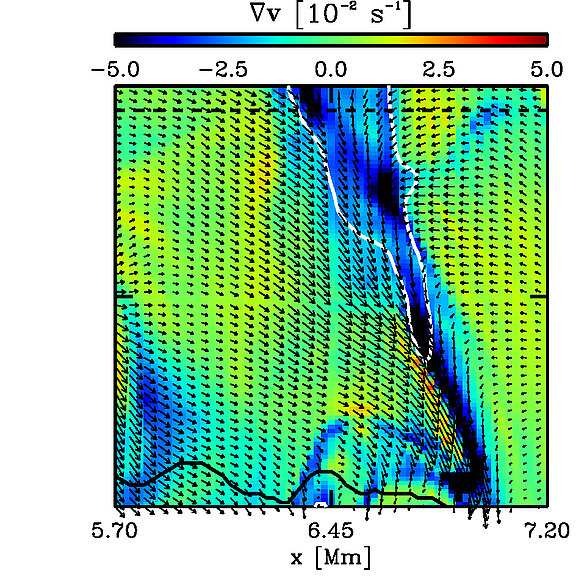
Magnetic flux emergence and cancelling in the quiet Sun is a frequently observed phenomena. The two possible physical flux removal mechanisms involved in its disappearance are retraction and reconnection. In order to find distinct observational signatures characterising retraction and reconnection we carry out three dimensional nongrey radiative magnetohydrodynamic simulations of convection near the solar surface and the solar photosphere using the STAGGER code, and employing different initial conditions: 1) mixed polarity simulations with alternating horizontal stripes of opposite vertical magnetic field and separated by a zero field stripe, and 2) flux emergence simulations with continuous injection of magnetic flux from the lower boundary. These initial conditions are meant to represent two different situations in the solar photosphere: magnetic flux cancelling in the absence or presence of magnetic flux emergence, respectively. We analyse the observational signatures of magnetic flux removal processes for flux emergence as well as for mixed polarity magnetohydrodynamic simulations. In the flux emergence simulation we are able to identify ubiquitous reconnection events anywhere from the solar surface to the upper photosphere. For a few of those reconnection events we can identify supersonic upflow velocities in the upper photosphere as well as strong temperature enhancements. Very close to the locations where reconnection takes place we also see strong electric currents, as well as supersonic horizontal velocities leading to sideways plasma compression. In the mixed-polarity simulations we only detect observational signatures of magnetic field retraction, related to large downflow velocities that appear in between regions where opposing horizontal velocities converge. These horizontal velocities are oftentimes supersonic, leading to heating due to shock dissipation. We do not see clear signatures of magnetic reconnection in these mixed polarity simulations. An example of retraction of magnetic field lines and its associated is presented on the right. We suggest that in the quiet Sun emerging flux regions the main flux removal process is reconnection, while in non flux emerging regions, retraction is the the dominant flux removal process.
Reference: Thaler, I. & Borrero, J.M. (2023), A&A, 673, A163
The magnetic field at the boundary between umbra and penumbra
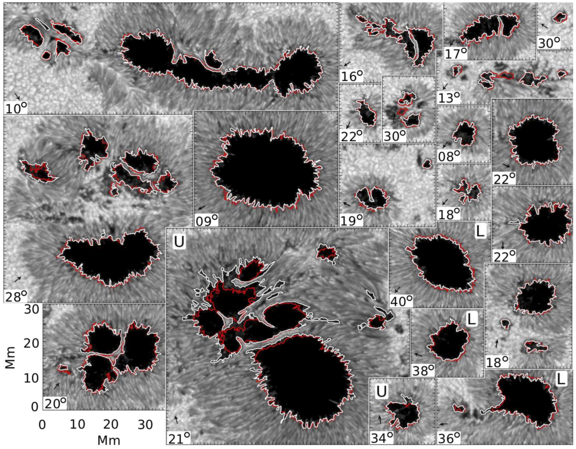
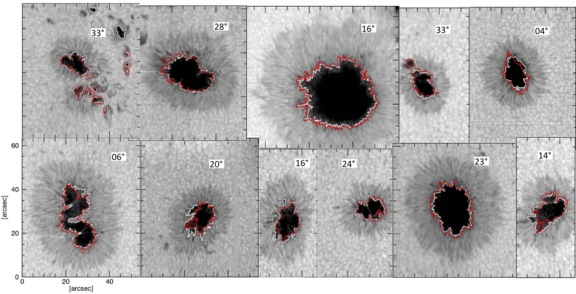
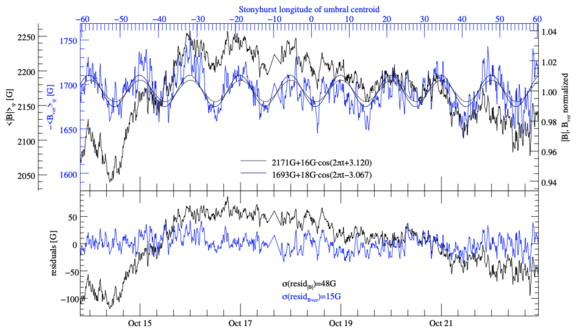
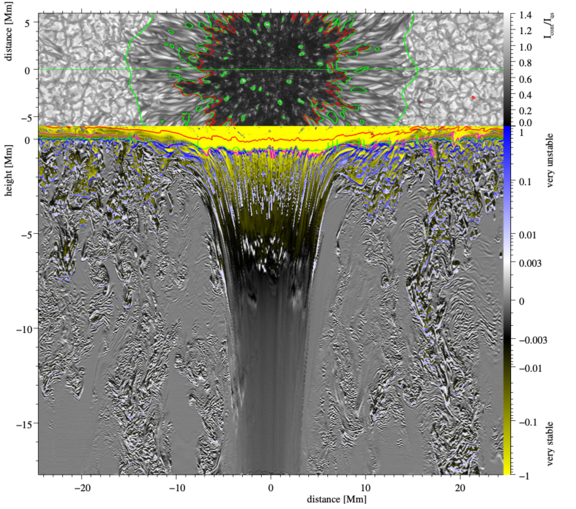
Sunspots are the longest-known manifestation of solar activity and their magnetic nature has been known for more than a century. Despite this, the boundary between umbrae and penumbrae, the two fundamental sunspot regions, has hitherto been solely defined by an intensity threshold.
Using Hinode satellite data, we can give statistical proof of an empirical law governing the boundary between umbra and penumbra in stable sunspots: The vertical component of the magnetic field, Bver, is invariant.
Contours of intensity at 50% of the granular quiet-sun value and contours of Bver=1867 G match. This is illustrated in the Fig. 1, in which contours for intensity (white) and Bver (red) are displayed for some of the spots that where included in the sample. Deviations were studied and shown to be non-significant (Jurcak et al. 2018).
This empirical law applies for all sunspots of the sample with different sizes, morphologies, evolutionary stages, and phases of the solar cycle. Thereby we have unveiled the magnetic property that discriminates between the umbral and penumbral modes of magneto-convection. This discovery carries strong consequences for the understanding of the fundamental processes of energy transport occurring in the magnetic Sun.
The validity of this empirical law and the value of this new constant is now being examined for different wavelengths and data sources. Figure 2 shows intensity images of sunspots observed with the GRIS@GREGOR spectograph. The value of Bver=1843 Gauss is consistent with the results from Jurcak et al 2018 with Hinode data.
We also studied the temporal evolution of Bver at the umbral boundary, in this case using HMI/SDO data. During the first disc passage, NOAA AR 11591, Bver remains constant at 1693 G with a root-mean-square deviation of 15 G, whereas the magnetic field strength varies substantially (mean 2171 G, rms of 48 G) and shows a long-term variation as shown in the residual in Fig. 3 after daily variations due to the satellite orbit are corrected. Hence, during the disc passage of a stable sunspot its umbral boundary can equivalently be defined by using the continuum intensity Ic or the vertical magnetic field component Bver. Contours of fixed magnetic field strength fail to outline the umbral boundary (Schmassmann et al. 2018).
Now, since observations have shown that in stable sunspots the umbral boundary is outlined by a critical value of the vertical magnetic field component, the key question is: What is the nature of the distinct magnetoconvection regimes in the umbra and penumbra? This is still unclear. To follow up on this, we analysed a sunspot simulation in an effort to understand the origin of the convective instabilities giving rise to the penumbral and umbral distinct regimes. We applied the criterion from Gough & Tayler (1966, MNRAS, 133, 85), accounting for the stabilising effect of the vertical magnetic field to investigate the convective instabilities in a MURaM sunspot simulation. The result is shown in Fig. 4: The deep subphotospheric sunspot trunk is magneto-convectivly stable. Upwards from some -5 Mm, columns of instabilty characterise the umbra of the sunspot. The subsphotospheric penumbra exhibits filamentary instabilities that are almost parallel to the solar surface. Hence, the Gough and Tayler criterion as a diagnostic tool reveals the tripartite nature of sunspot structure with distinct regimes of magneto-convection in the umbra, penumbra, and granulation operating in realistic MHD simulations. (Schmassmann et al. 2021)
References:
- Jurčák, J., Rezaei, R., Bello González, N. Schlichenmaier, R., Vomlel, J., 2018, Astronomy & Astrophysics, Volume 611, L4
- Schmassmann, M., Schlichenmaier, R., Bello González, N. 2018, Astronomy & Astrophysics, Volume 620, A104
- Lindner, P., Schlichenmaier, R., Bello González N., 2020, Astronomy & Astrophysics, Volume 638, A25
- Schmassmann, M., Rempel, M., Bello González, N., Schlichenmaier, R., Jurčák, J., 2021, Astronomy & Astrophysics, Volume 656, A92
Data-constrained 3D modeling of a solar flare evolution
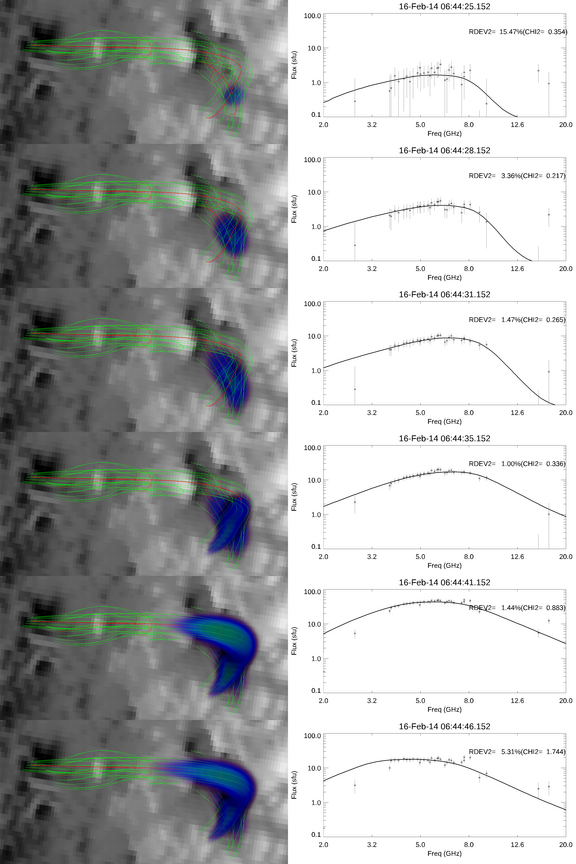
Solar flares are driven by release of the free magnetic energy and its conversion to other forms of energy–kinetic, thermal, and nonthermal. Quantification of partitions between these energy components and their evolution is needed to understand the solar flare phenomenon including nonthermal particle acceleration, transport, and escape and the thermal plasma heating and cooling. The challenge of remote sensing diagnostics is that the data are taken with finite spatial resolution and suffer from line-of-sight (LOS) ambiguity including cases when different flaring loops overlap and project one over the other. We address this challenge, for the first time, by devising a data-constrained evolving 3D model of a moderate solar flare. We developed a 3D magnetic model consistent with available imaging data and constrained by the underlying photospheric magnetic field. Then, for each time frame, we adjusted the distributions of the thermal plasma and nonthermal electrons in the model, such that the observables synthesized from the model matched the observations (see figure/animation). This approach removes the LOS ambiguity and permits to disentangle contributions from the overlapping loops. It reveals new facets of electron acceleration and transport, as well as heating and cooling the flare plasma in 3D. We find signatures of substantial direct heating of the flare plasma not associated with the energy loss of nonthermal electrons.
Reference: Fleishman, G.D., Nita, G.M., Motorina, G.G. "Data-constrained 3D modeling of a solar flare evolution: acceleration, transport, heating, and energy budget", ApJ; (in press)