Research Areas of the Solar-Stellar Connections Group
We study solar and stellar magnetism observationally and through MHD simulatons. Our goal is to amalgamate the understanding of magnetism in cool late-type stars into a unified dynamo paradigm that encompasses stars of different masses and ages. The wealth of detailed data we have for the Sun is of vital importance for this research, whereas data from other stars helps to put the Sun into its proper place in the bigger picture of stellar activity. This research is also connected to the influence of stellar radiation and activity on atmospheres and habitability of exoplanets.
For this research, we employ the polarized radiation transport theory for the continuum, atoms and molecules, MHD simulations on various scales ranging from simplified turbulent cubes to local simulations of solar and stellar atmospheres and to global simulations covering several solar and stellar dynamo cycles, spectropolarimetric measurements for the Sun and stars, broad-band polarimetric measurements for exoplanetary atmospheres, laboratory and airborne spectropolarimetric measurements of various natural and artificial samples and environments.
Global simulations of stellar magnetism
We use the Pencil Code to make global and semi-global MHD simulations of stellar dynamos. The Pencil Code is a free versatile finite-difference solver for ordinary and partial differential equations. In the research of global dynamos we use it in two configurations:
1. Semi-global spherical wedge geometry where the polar caps are cut out and often only a quarter of the longitude is modeled as a compromise between realistic geometry and globality.
2. Star-in-a-box setup where a spherical star is embedded into a Cartesian cube, avoiding coordinate singularities of spherical polar coordinates and allowing simulations of full stars including the centre.
The former setup has recently been used to study differential rotation near the transition from anti-solar (slow equator, fast poles) to solar-like (fast equator, slow poles) rotation profile (Käpylä 2023). This study confirmed earlier results regarding the Prandtl number (Pr) dependence of differential rotation for Pr > 1, but indicated that such dependence vanishes for Pr < 1 which is the regime relevant for the Sun. The influence of magnetism and higher levels of turbulence in terms of the Reynolds number were deemed more important. Furthermore, the angular momentum transport leading to the
generation of differential rotation was shown to originate from large-scale convection, also known as banana cells or Busse columns, which have not been detected in the Sun.
The star-in-a-box simulations have been used to study magnetism in fully (Käpylä 2019, Ortiz-Rodríguez et al. 2023) and partially convective stars (Käpylä 2022). The studies of fully convective models indicate that the global dynamos of fully convective stars depend on the rotation of the star and the Reynolds and Prandtl numbers similarly as in earlier spherical shell simulations. This can be interpreted such that the dynamos in simulations of fully and partially convective stars are driven by the same processes irrespective of the geometry. Furthermore, the partially convective models with the star-in-a-box models yield cyclic solutions quite like those of the Sun. Such solutions appear to be easier to excite than in spherical shell models. A possible explanation is that the magnetic field at the stellar surface is more free to evolve in the star-in-a-box simulations -- where no hard surface boundary is present -- as opposed to spherical shells where a boundary condition -- that is often unrealistically strict -- is imposed.
References:
Molecules in Magnetic Fields
A series of theoretical works on quantum mechanics of molecules in magnetic fields have established a new field in astrophysics and created a vast potential for exploring magnetic fields and unresolved spatial inhomogeneities in cool astrophysical environments and have led to several discoveries in solar and stellar magnetism. We possess now the following theoretical tools:
- A numerical approach for calculating the Zeeman effect in diatomic molecules for the intermediate momenta coupling case for terms of any multiplicity (Berdyugina & Solanki 2002);
- Theory of polarised radiative transfer for molecular lines in the presence of magnetic fields in stellar atmospheres and a systematic analysis of diagnostic capabilities of molecular lines for studying the magnetic and thermal structure of sunspots, starspots, and cool stars and substellar objects (Berdyugina et al. 2003);
- Theory of polarised scattering of radiation by molecules and explanation of the enigmatic behaviour of molecular scattering polarisation in the solar spectrum (Berdyugina et al. 2002);
- Theory of the molecular Paschen-Back effect for different momenta coupling cases (Berdyugina et al. 2005);
- Theory of the molecular Hanle effect in the Paschen-Back regime (Shapiro et al. 2006).
This research continues to find new ways for resolving problems in solar and stellar magnetism.
Magnetism of Cool Stars
Using a novel Doppler Imaging (DI) technique for mapping stellar surfaces based on the advanced inversion method called Occamian approach (Berdyugina 1998), we obtained time-series of temperature maps of very active stars and investigation of spot evolution and stellar activity cycles (Berdyugina et al. 1998, 1999, 2000; Berdyugina & Marsden 2006; Järvinen et al. 2007, 2008; Korhonen 1999, 2008). Based on these DI results and long-term photometric records, we discovered permanent active longitudes and a new type of activity cycles (flip-flop cycles) on very active binary and single stars (Berdyugina & Tuominen 1998; Berdyugina et al. 2002; Korhonen et al. 2002; Järvinen et al. 2005ab). To explain such an unusual ocsillatory behaviour of global stellar magnetic fields we proposed a novel interpretation of stellar activity cycles in terms of dynamo modes (Fluri & Berdyugina 2004). In addition, we recovered the first stellar butterfly diagram on a very active cool star (Berdyugina & Henry 2006) and detected for the first time molecular polarisation from unresolved starspots (Berdyugina et al. 2006). The first 3D Zeeman-Doppler image of an M dwarf was obtained from simultaneous inversions of atomic and molecular Stokes profiles (Berdyugina 2011) and the complexity of magnetic fields in the atmospheres of M dwarfs was found dependent on the stellar mass and with the height in the atmosphere (Afram & Berdyugina 2019). |
Magnetism of Brown Dwarfs
Recently, we have detected for the first time a strong magnetic field on a brown dwarf exhibiting transient non-thermal radio and optical emission bursts (Berdyugina et al. 2017; Kuzmychov et al. 2017). We have measured near-infrared polarized and optical emission spectra of LSR J1835+3259 at several aspect angles during its two rotational periods of nearly 3h on two consecutive nights. During the first night, the magnetic field is found to be at least 5.2 kG and cover at least 11% of the dwarf visible hemisphere. This is first time that we can quantitatively associate brown dwarf non-thermal bursts with a few kG surface magnetic field and solve the puzzle of their driving mechanism. The emitting region topology recovered using spectral line profile inversions indicates the presence of hot plasma loops of at least 7000K with a vertical stratification of the sources producing both optical and radio emission. These loops rotate with the dwarf in and out of view causing the emission bursts. The 5 kG magnetic field is detected at the base of the loops, while different frequency radio bursts are found to be associated with hydrogen optical emission sources at different heights above the surface where the magnetic field is weakens first to 2.5-2.8 kG at the H-beta height, then to 1.5-1.9 kG at the H-gamma height, and finally to 1.5-1.6 kG further above. We concluded that the activity on LSR J1835+3259 is probably driven by an interaction of a large scale magnetic field (active regions or magnetic poles) with small-scale, entangled, wide-spread and rapidly evolving magnetic fields. Their evolution leads to reconnections, flares, aurora-like emission, and radio-bursts modulated by the fast rotation. Our detection provides the first direct observational constraint for a magnetically driven non-thermal emission mechanism and for generation of magnetic fields in fully convective ultra-cool dwarfs. It also paves a path towards magnetic studies of hot Jupiters of similar temperatures.
Magnetism of White Dwarfs
White dwarfs are end products of the evolution of stars like the Sun. About 20% of them are observed with strong magnetic fields. We investigate their magnetism using spectropolarimetric measurements in molecular bands and the continuum. In particular, we obtained the first detailed modeling of broad-band polarization in such molecular bands like CH and C2 of the unique magnetic white dwarf G99-37 and obtain its global magnetic field of 7 MG (Berdyugina et al. 2007). This white dwarf has been unique for about four decades because it features the strong circular polarizion in the blue CH bands of G99-37 discovered in the 1970s. By carrying out a spectropolarimetric survey of cool white dwarfs with molecular C2 bands, we have discovered the second magnetic white dwarf with the CH molecular bands which were hidden in the absorption of the C2 bands (Vornanen et al. 2010). The magnetic field of this white dwarf was estimated to be about 2 MG. Now, we undertake a new survey of white dwarfs without spectral features to detect their magnetic fields via circular polarization in the continuum radiation with our new polarimeter DIPol-UF (Piirola et al. 2020). This survey has already resulted in detection of two new magnetic white dwarf (Berdyugin et al. 2021). Using these novel approaches, we expect to increase the fraction of known magnetic white dwarfs.
Small-scale Magnetism of main-sequence Stars
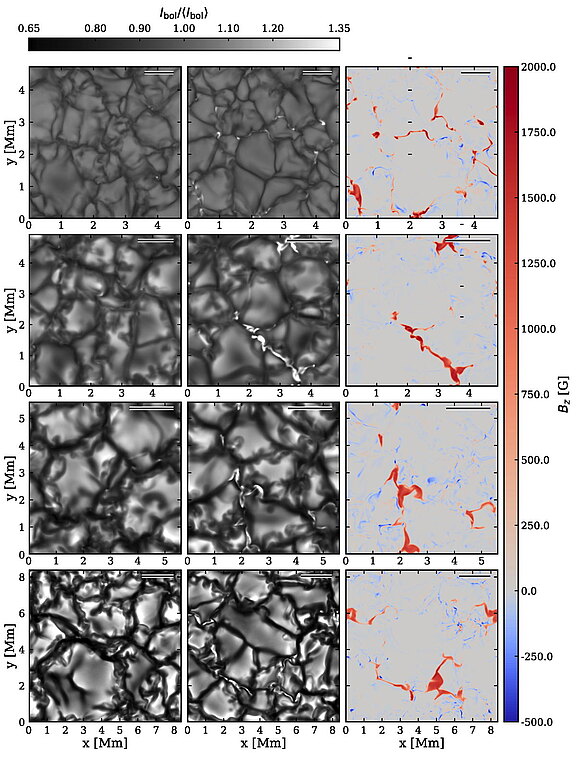
I the past, we carried out numerical simulations of small-scale magnetic fields in main-sequence stellar atmospheres of spectral types from F5V to K8V (Steiner et al., 2014; Salhab, Steiner, Berdyugina et al., 2018). We discovered (i) that the presence of small-scale magnetism increases the bolometric intensity and radiative flux in all the investigated spectral types, but maximally so for solar analogues, (ii) that the field strength at visible optical depth τc = 1 stays approximately constant, independent of the spectral type, and (iii) that the field strength is close to (thermal) equipartition for the Sun and spectral type F5V but stays clearly sub-equipartition for K2V and more so for K8V. The main conclusion is that small-scale magnetism produces a positive contribution to the bolometric luminosity of stars in the spectral range from F5V to K8V. Since the amount of small-scale magnetism changes with the solar or stellar cycle, we expect a corresponding modulation in the solar or stellar luminosity. The modulation is most effective for early G-type stars. In a next step, we adjust the stellar parameters to closer match the main sequence, add M spectral types, and plan to study and simulate the origin of these magnetic fields. Simulating stellar atmospheres other than that of the Sun helps us to better understand the Sun and to place it in context with other stars. (O. Steiner with F. Riva (IRSOL) et al.).
What are the effective Viscosity and Resistivity of numerical Simulations?
A crucial step in many numerical investigations of magnetohydrodynamics consists in an accurate determination of the magnetic Prandtl number, Prm. But for this purpose one must determine the effective viscosity and plasma resistivity intrinsic to the simulation. Presently, we develop a reliable methodology for estimating the effective viscosity and resistivity and hence, the Reynolds and magnetic Reynolds numbers, Re and Rem, and their ratio Prm = Rem/Re. We plan to apply this methodology to numerical simulations of the turbulent small-scale dynamo that supposedly generates the bulk of small-scale magnetism on the solar surface (O. Steiner with F. Riva (IRSOL)). The development of numerical methods for the simulation, post-processing, and code validation is an important and continuous part of our research work.
Vortex Motions and magnetic Tornadoes on the Sun
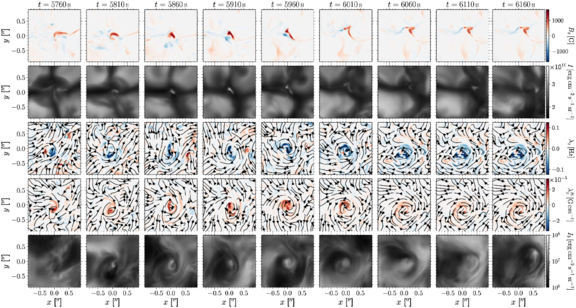
With the advent of high-resolution observations of the solar atmosphere with modern solar telescopes and post-focus instruments, it became clear that there exist many forms of vortical motions in the solar atmospheres not unlike to the terrestrial atmosphere where they appear in the form of hurricanes or tornadoes. Also in the solar atmosphere exist different types of vortical motions. In the past, we simulated and predicted the existence of non-magnetic bright points that occur as a consequence of swirling downdrafts in the intergranular space at the visible solar surface (Calvo, Steiner & Freytag, 2016). These swirls are so tiny that they escaped detection in the past but the simulations suggest that they should become visible with the upcoming 4-m class solar telescopes. Once sufficient magnetic flux is accreted by such a swirling downdraft, the vortical motion is mediated to the outer atmosphere where it can be observed as chromospheric swirl.
Recently, we numerically simulated solar magnetic tornadoes and found that chromospheric swirls were the visible signature of torsional Alfvén pulses that propagate from the photosphere up into the chromosphere (Battaglia, Canivete Cuissa, Calvo, Bossart & Steiner, 2021). But what exactly causes these pulses and what is their fate in the outer atmosphere? Do they contribute to heating the outer atmosphere and in which way and how much? These questions are subject of further research. Currently, we are developing a new method for the accurate identification of swirling motions in data from simulations and observations (O. Steiner with R.-J. Canivete Cuissa (IRSOL)).
Space Weathering of Rocky Planets
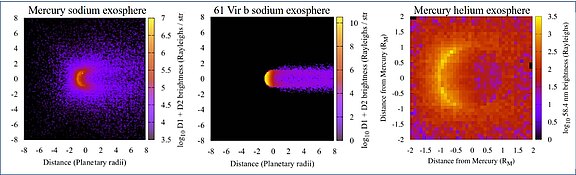
Rocky exoplanets are expected to be eroded by space weather in a similar way as in the solar system. In particular, Mercury is one of the dramatically eroded planets whose material continuously escapes into its exosphere and further into space. This escape can be traced by atoms such as sodium and helium, scattering sunlight. Due to solar wind impact, micrometeorite impacts, photo-stimulated desorption and thermal desorption, atoms are released from surface regolith. Some of these released atoms are escaping from Mercury's gravitational-sphere. They are dragged anti-Sun-ward and form a tail structure.
We have simulated space weathering of Mercury by escaping sodium atoms (Yoneda et al. 2017) and helium atoms (Yoneda et al. 2021). The Mercury exosphere in the optical Na I doublet was previously detected in ground-based observations, while in the ultra-violet He I line it may be detected with space missions. The escape of helium atoms from Mercury was found to be sensitive to the solar helium line profile, and this can be verified with space data.
We expect similar phenomena on exoplanets. For example, the hot super-Earth 61 Vir b orbiting a G3V star at only 0.05 AU may show a similar structure. Because of its small separation from the star, the sodium release mechanisms may be working more efficiently on hot super-Earths than on Mercury, although the strong gravitational force of Earth-sized or even more massive planets may be keeping sodium atoms from escaping from the planet. For 61 Vir b, we found (Yoneda et al. 2017) that sodium atoms can escape from this exoplanet due to stellar wind sputtering and micrometeorite impacts to form a sodium tail. However, in contrast to Mercury, the tail on this hot super-Earth is strongly aligned with the anti-starward direction because of higher light pressure. An exo-base atmosphere on 61 Vir b seems similar to that of Mercury.
Exoplanet Topography, Biosignatures, and artificial Mega-structures
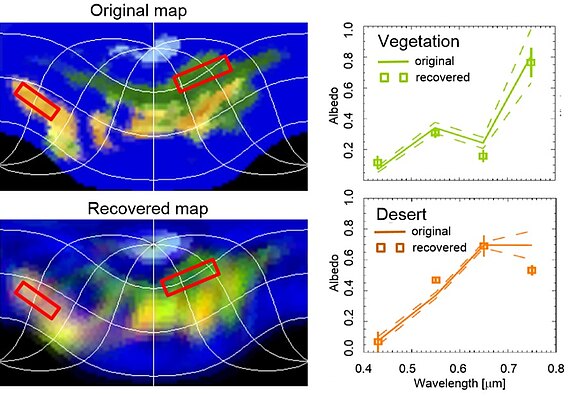
Seeing oceans, continents, quasi-static weather, and other surface features on exoplanets may allow us to detect and characterise life outside the solar system. The Proxima b planet resides within the stellar habitable zone allowing for liquid water on its surface and it may be Earth-like. However, even the largest planned telescopes will not be able to resolve its surface features directly. In a recent paper (Berdyugina et al. 2019), we demonstrated an inversion technique to image indirectly exoplanet surfaces using observed unresolved reflected light variations over the course of the exoplanets orbital and axial rotation: ExoPlanet Surface Imaging (EPSI). We showed that the reflected light curve contains enough information to detect both longitudinal and latitudinal structures and to map exoplanet surface features. We demonstrated this using examples of Solar system planets and moons as well as simulated planets with Earth-like life and artificial megastructures. We develop this techniques further to include polarimetric measurements.
PhD Project: Sunspot Penumbral Filaments
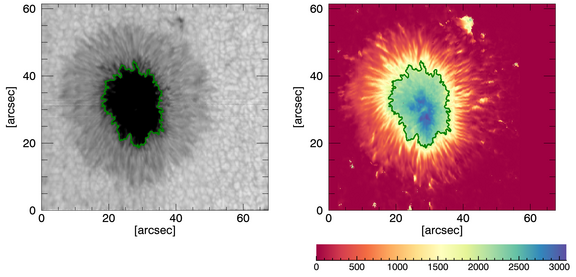
Sunspots the most prominent manifestations of magnetic fields on the sun. While it has been clear for many years that the dark inner part of a sunspot (the umbra) harbors strong vertical fields of up to 3000 Gauss and the outer filamentary part (the penumbra) harbors inclined fields of intermediate field strengths, it is still only poorly understood how and under which circumstances sunspots and especially the penumbra form. Using high resolution data from the best solar telescopes of the world, we are working on revealing the mechanisms of this formation. By observing different lines with spectropolarimetric instrumentation, we access information about the atmospheric conditions at different atmospheric heights in the photosphere and in the chromosphere. Stokes inversion tools allow us to extract models of the three-dimensional topolography of the magnetic field vector, the temperature and the velocity from the spectropolarimetric data. We then study the temporal and spatial evolution of these physical parameters to find mechanism and conditions under which, e.g. the penumbra forms or discriminate between existing hypothetical formation scenarios. This project is part of the PhD thesis of Philip Lindner.
PhD Project: Short-lived small-scale solar magnetic Fields
Quiet sun regions in the solar atmosphere harbour short-lived small scale magnetic structures. Magnetic elements in the internetwork region show high dynamic, evidencing processes such as flux cancellation, convective collapse or granular buffeting, which play a role in the magnetic flux exchange with the upper atmosphere. This project focuses on the statistical analyses of such magnetic structures in order to characterise them and search for possible correlations with atmospheric parameters such as local heating. Taking advantage of multi-instrument data sets and novel techniques in machine learning and computer vision, a comprehensive picture of these processes from the photosphere to higher layers of the solar atmosphere will be achieved. This research is carried out in the framework of the QUEST project and is part of the PhD project of Saida Díaz Castillo.